No more strep throat
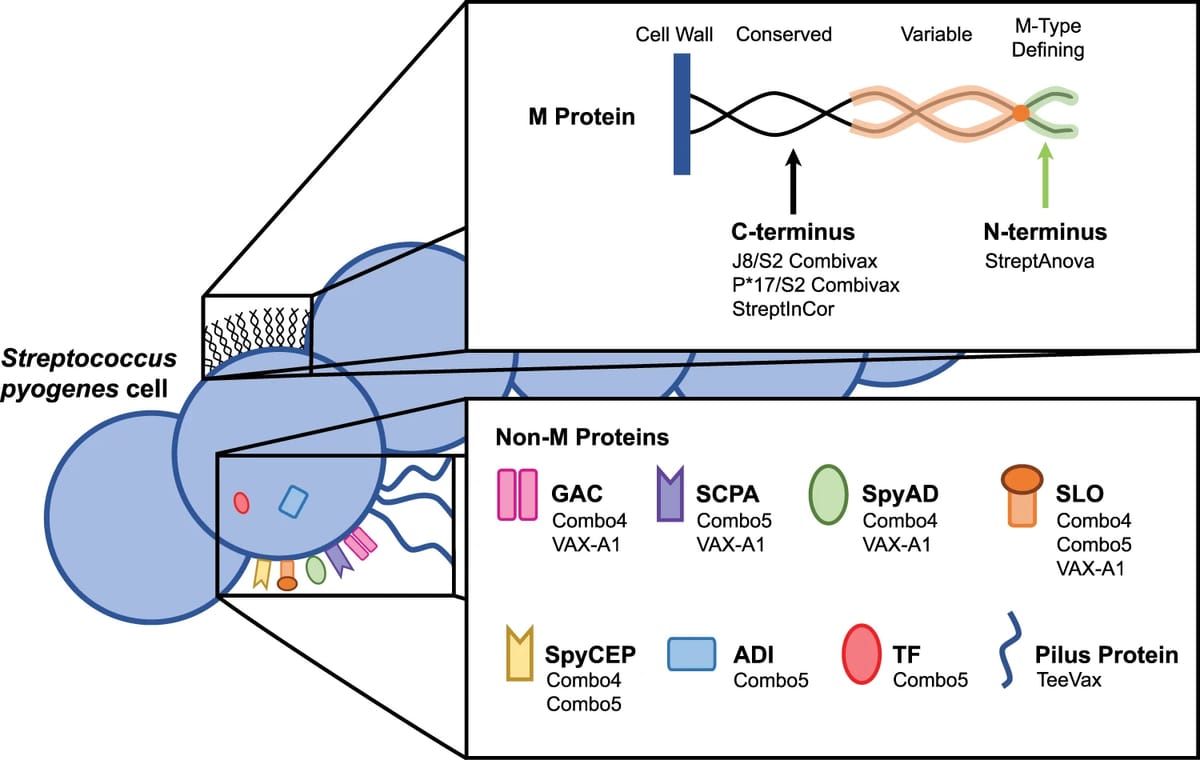
I last got strep throat the first month out of lockdown. I had been indoors, in public, and was being punished. It was cruel and unusual to check me just when we were allowed to enter the world again. I went to my doctor with three negative COVID tests to insist I was simply sick – normal sick. He swabbed my tonsils, swilled the stick in a liquid container, and snapped off the stem. It felt exotic, at least, to be the subject of a test for something else entirely, something I’d forgotten about – though the cartridge’s lines showed the familiar pink, as the liquid crept up the paper. Then one red line, then two. My doctor said he’d never seen such a clear positive result, so quickly. I always take pride in my achievements. I was out of there, trying to hold my breath and coughs in line for amoxicillin at Walgreens.
I do not harbour a grievance that I was never offered a vaccine against strep as a kid, I promise you. But I do have questions. My sickness in 2021 was fine after the antibiotics, just annoying. I didn’t work for a bit, and I went back to being a recluse. It is not so trivial for many parents. Last month, my colleague’s four year old came down with strep, taking her and her husband down too. It was stressful:
They ended up going to urgent care in case, since infections can damage your kidneys or turn deadly if the bacteria spreads through your blood – invasive disease. Happily, that’s rare; sadly, invasive toddler infections have been increasing recently not just in the U.S. but in Canada, the U.K., and Japan – so I understand the fear.
(Even in the simpler cases, where no one’s life is at risk, I wonder – if I’m on antibiotics, and my colleagues are on antibiotics, and their kids are on antibiotics, how long will those drugs last before the bug becomes resistant?)
I was not told this growing up, but strep A kills more people than brain cancer. Repeated untreated infections are bad for you. They can lead to rheumatic fever, and eventually rheumatic heart disease. That is uncommon in rich countries these days, but common in many lower- and middle-income countries.
That means two of the big technology questions our generation faces are: who will make the first strep A vaccine? And: who will make the best one? Fixes for the other big killers are often elusive, but scientists already know quite a bit about strep A bacteria. A vaccine should be possible. None is close to the finish line.
You will have to remember some high school biology to read this post, but you don’t need any technical background beyond that. I’ve put some of the terminology you’d see in scientific papers in parentheses, so that you can search around further using them if you want.
Proteins and carbohydrates
With any good vaccine, you’re trying to trigger a lasting immune response. I'm about to talk about linking (conjugating) a carbohydrate from strep A bacteria to a protein from either strep A or another microbe. Keep in mind the purpose all along is about lasting memory. Six paragraphs first and then we'll get there…
There are many ways to make a vaccine, and the oldest school way is take the pathogen and weaken it then inject it (“live attenuated vaccine”). That’s not a great option for Strep A because some human tissue looks similar to some bits the bacteria (“molecular mimicry”), which can trigger your immune system to attack your heart valves (“cross-reactivity”, “autoimmunity”) leading to rheumatic fever -> rheumatic heart disease. When designing a strep A vaccine, scientists don’t want to trigger some parts of the immune system.
A more common modern development method, that's often safer, is (1) figure out which bits of the pathogen are important to its survival + exposed to your immune system (antigens), and are found in strains of the pathogen globally so everyone can benefit, (2) put those bits on a plate with some immune cells/antibodies – then give them to an animal who's later exposed to the pathogen, then eventually a person – to find out which work as vaccines (this step will take a few iterations...), then (3) grow up only those bits in a biological system like E. coli or yeast or insect cells or the bacteria itself (if the pathogen is a bacteria) in a series of increasingly large vats, harvest the parts you want, purify them down, and inject them (“recombinant DNA”; “biotechnology”; also see “synthetic biology”). There are other steps too, e.g. sometimes adding an adjuvant, making a solution, putting it in vials, freeze-drying – but that's the gist. This is the main way you'd make a “subunit” vaccine, i.e. where you're using a part or parts of the pathogen. (Ignore mRNA vaccines for now. They typically code for your cells to make a subunit of the pathogen, e.g. SARS-CoV-2’s spike protein. Making mRNA vaccines is very different though; you usually don't need any cells let alone living replicating ones.)
Sometimes these subunits are proteins – e.g. the Novavax COVID vaccine is made by cloning the gene that encodes the spike protein into a virus (baculovirus) then infecting moth cells with that virus, so the moth cells display the spike protein on their surface and you can harvest 'em. The RTS,S and R21 malaria vaccines are made by writing a long string of DNA that codes for a fused fandango of (a) part of the malaria circumsporozoite protein and (b) the hepatitis B surface antigen, then cloning that string into a circle of DNA (a “plasmid”), introducing that plasmid into yeast cells (introduction = “transformation”) where it gets transcribed (DNA->mRNA) and translated (mRNA->proteins) into proteins that cleverly self-assemble to look kind of like a virus ("virus-like particle", VLP), then harvesting and purifying the VLPs. (The hep B antigen is partly there to make the self-assembly happen so that lots of circumsporozoite proteins get displayed to your immune system – scientists, AKA GSK and my now-colleague Katharine Collins, borrowed that function from evolution; the hep B virus uses its surface antigens to get into your liver cells, then makes more of those antigens when leaving again, some of which self-assemble into particles.)
For vaccines against bacteria, the subunits are sometimes carbohydrates too – e.g. the pneumococcal vaccines that Gavi buys are PCV10 and PCV13, which contain 10 or 13 polysaccharides from the outer capsule of different strains of pneumococcus bacteria. You grow those by letting 13 strains of pneumococcus proliferate in 13 bioreactors, then isolating the polysaccharides from each. You'll see “carbohydrate”, “sugar”, “glycan”, “surface glycan”, “peptidoglycan”, “polysaccharide”, and “capsular polysaccharide” all used in bacterial vaccine discussions; they're related and you can ask ChatGPT about them if you're interested in more detail. For strep A, “surface glycan” ends up being important, because...
Strep A has one main carbohydrate called GAC, exposed on its surface, that’s shared by many evolutionary brethren/strains of the bacteria (“conserved”). Indeed the way different Streptococcus groups were originally defined was by those surface glycans (the Lancefield system). GAC stands for Group A Carbohydrate. Talk about conserved! There are also a bunch of proteins the strep A research community expects are antigens: the M protein, SpyCEP, SpyAD, SCPA, SLO, ADI, TF, and the Pilus Protein. Time to go back to that diagram:
Today, scientists are studying those antigens in more depth – but in previous generations it was not easy to make a career out of strep A. The FDA banned vaccine trials in 1979, until 2006, chilling the field. Funding came in dribs and drabs. In the last five years, public funders have started poking around. That includes Open Philanthropy, where I work; we started funding vaccine development work in 2020. Since then we’ve made some other grants, including supporting a professor in Australia to take 5 of those proteins in combination – ADI, SCPA, SLO, SpyCEP, and TF – through non-human primate trials with different adjuvant combinations. Those experiments will teach the research community how well each adjuvant works with that combination of 5, and also help (in)validate how predictive/useful the mouse model for strep A is, since that academic group already has results in mice. Meanwhile in Italy, GVGH – the global health arm of GSK – is working on “Combo4” from the antigen diagram. Combo4 consists of GAC conjugated to a (detoxified/edited) toxin from diphtheria called CRM197, in combination with 3 other strep A protein antigens – SLO, SpyCEP, and SpyAD.
O.K., I just said “conjugated to a (detoxified/edited) toxin from diphtheria called CRM197”. Well done on surviving the six paragraphs and making it to conjugation! Why add in a toxin rather than just inject GAC itself, and why a diphtheria toxin? You may remember, it's about memory. Carbohydrate antigens trigger B cells to produce antibodies (good), but what you ideally want is a “T cell dependent” response – where antigens also trigger CD4+ T helper cells that in turn trigger B cells -> antibodies. That's important for all of our immune memories, but especially infants’. Proteins tend to be better than carbohydrates at stimulating that T cell response – so glycoconjugate vaccines link your carbohydrate (“glyco”/glycan) of interest (in this case GAC) to a protein. That doesn't have to be a protein from the same bacteria. Detoxified-but-still-immunogenic versions of toxins from tetanus (TT) and diphtheria (DT and CRM197) are the most commonly used so far. They've been a big deal for 21st century babies.
Sure enough, the 10 or 13 polysaccharides I mentioned in pneumococcal vaccines are each conjugated to a carrier protein, which is why those newer vaccines work well. For PCV13, the carrier for each of the 13 is CRM197; for PCV10 it's a mix of TT, DT, and a protein from Haemophilus influenzae (Protein D). Typhoid is caused by bacteria; the new typhoid vaccine I blogged about conjugates the “Vi” polysaccharide from those bacteria with DT. At Open Phil we’re also co-funding a conjugate cholera vaccine trial, alongside the Korean RIGHT Foundation, combining the “O-”polysaccharide from cholera with TT, because the existing oral vaccines don’t generate a great immune response in under-2s (though we love ‘em and are helping expand oral vaccine supply given all the recent cholera outbreaks).
So, in summary: scientists are testing strep A protein vaccines as well as combination carbohydrate-protein vaccines to see what works. They are also testing different adjuvants with those leads to boost the immune response, and are also “testing” the animal models themselves for how consistent mouse<>primate results are, and eventually mouse<>primate<>human.
Strep A vs tuberculosis vs syphilis
Or I should really say: Streptococcus pyogenes vs Mycobacterium tuberculosis (Mtb) vs Treponema pallidum (Tp). These are the three bacterial vaccines on my list of 10 lifesaving technologies that don’t exist yet. Here are four other bacterial vaccines already invented and in use, to turn to for comfort as you read the next section full of difficulties: Hib, typhoid, bacterial pneumonia, meningitis.
How does humanity’s collective state of knowledge, on topics relevant for vaccine development, compare between strep A, TB, and syphilis? Some of my colleagues who have made grants on each have more informed views on that question than I do, but here are mine to start. At a high level, my impression is TB has been studied the most, then strep A, then syphilis. Breaking that down into areas/fields:
- Vaccines in use: There is only one TB vaccine, first used in 1921; it's great as an infant vaccine but, with some asterisks, it is known not to work well in adults. There are no strep A or syphilis vaccines.
- Microbiology: Studies of each bug go back more than a century. They each have 1000-4000 genes, with some amount known about which genes contribute to virulence, antibiotic resistance, and so on. That said, syphilis seems like the dark horse of the three here, because until 2016, only 31 genomes of Tp had been sequenced – ever! – and until 2018 Tp could not be cultured in a dish – you needed a rabbit. In a recent study trying to fix the genome paucity problem, scientists swabbed people’s lesions to collect samples, took the stairs up to the hospital roof, and infected rabbits they kept up there so the rabbits could grow enough copies of Tp to sequence. That’s old school. Understandably, not having easy access to the microbe has made it difficult to run standard gene knockout/knockdown/overexpression/etc experiments to determine each gene’s function (“functional genomics”). TB has received perhaps the most attention over the years but research on the microbe itself has been hampered by risk to researchers – it’s a BSL-3 pathogen, meaning you have to take a variety of precautions to work with it, and there are only so many BSL-3 labs around.
- Structural biology: This is where you zoom in on particular features of interest rather than looking at the whole microbe – for example outer membrane proteins that could be vaccine targets. Techniques include x-ray crystallography, NMR spectroscopy, electron microscopy, and cryo-electron microscopy (“cryo-EM”). Once again I suspect Tp is behind, in part since it’s fragile, in part since the TprK protein on the outer membrane is variable – in part since the bacteria look different on their own vs glommed on to mammalian cells. I would guess Mtb is leading the pack in this category too, given it’s had much more drug development effort poured into it, but I don’t know.
- Pathogenesis: This refers to how the bacteria leads to disease. Again I assume TB, especially TB in the lungs (“pulmonary”), is the most studied – but that there are gaps, e.g. since granuloma formation makes disease progression harder to study in non-human animal models. Mice and guinea pigs form granulomas, but they look different to humans’. Strep A is complicated because it causes many different diseases rather than just one, and the biggest killer is rheumatic heart disease from autoimmunity rather than pathogenesis per se (“sequelae”). That said, the link between strep A and rheumatic fever/heart disease is well established and the drivers of autoimmunity relatively well understood. Tp moves all over the place too (unusually “motile”), including crossing the placenta, by burrowing through your tissues in a creepy spiral (“spirochete”); some forms of syphilitic disease are better understood than others.
- Immunology: In some sense you have to hand it to TB here since it already has an effective vaccine when the other two don’t. I don’t know who is winning on other more specific immunological questions, though I’d bet syphilis is losing. (Questions like: What mechanisms have the bacteria evolved to evade the immune system? Which antibodies neutralise the bacteria? What promotes a useful T cell response, and how important is that? How much does human genetic variation affect vaccine effectiveness? How important are adjuvants? Will oral or nasal delivery work? Etc.)
- In vitro models: This category overlaps with the microbiology and immunology ones above, but I’m thinking in particular of: what standardised tests can you run to see how well a vaccine candidate might work? Some classics are: neutralisation assays, growth inhibition assays (GIAs), opsonisation assays, cytokine production assays, ELISPOTs for quantifying T cell responses… Then you could also include organoids in this category – e.g. at Open Phil we’ve funded development of a tonsil model for TB. Tp hasn’t had a growth inhibition assay until recently, since it’s only recently been growable, but at least it’s a Biosafety Lab 2 pathogen, as is strep A. As mentioned, TB experiments rightly have to be done in BSL-3 conditions, to prevent researchers from getting infected. This makes progress in the field slower and more expensive.
- Animal models: The syphilis field works with rabbits, occasionally rhesus macaques, and recently mice. TB works with mice, guinea pigs, and primates. Strep A goes for mice and rats, and occasionally primates – but that’s for acute infection, rather than rheumatic heart disease, which is harder to study in animals. Rabbits have done a lot for syphilis (they develop lesions and mice don’t), but I’ve heard people complain about every one of these animal models, for different reasons. Room for improvement across the board.
- Clinical research models: This means clinical trials for safety and efficacy, but I’d also include pharmacology, host genetics, and immunogenicity under this heading. TB trials mostly go for prevention of pulmonary disease as a primary endpoint, and those trials take years and cost gazillions because TB disease is low incidence so you get hardly any events in the control or treatment arm (happy news for patients, of course). Strep A causes pharyngitis, which is easy to power a trial against because it’s so high incidence basically everywhere; rheumatic fever and risk of rheumatic heart disease as endpoints would be harder, though, and regulators may request more safety data than usual given autoimmunity concerns. Syphilis, who knows.
- Epidemiology: I remain astonished how little is known about the incidence and prevalence of infection and disease for all three of these. TB is especially !, given it kills over a million people a year; researchers don't know whether billions of people are latently infected right now or "only" hundreds of millions. That said, syphilis and rheumatic heart disease take the cake in that even their mortality burden estimates might be 2 times off the truth globally and I wouldn’t be that surprised.
There are other fields that are relevant to developing vaccines against these three bugs, that vary between the bugs, which I haven’t given a bullet of their own since this post is long already: manufacturing, health economics and modeling, implementation strategies/public health, post-licensure pharmacovigilance/monitoring requirements, “real world evidence”, etc.
How do these bugs compare to bacteria that have approved vaccines already? Were there points in the development of those vaccines that felt kind of “like” where we’re at now for strep A? Or have the tools available to bacteriologists improved so much since then that any analogies are strained? I don’t know. I’d love to learn where each of those vaccines got stuck in development, before they were available, and what unlocked progress. Better to know soon for, one day, I may have kids.
Thank you to George Rugarabamu, who first pointed out to me that rheumatic heart disease is one of the most neglected fields relative to its importance in modern biomedicine, when we were in Ethiopia for an unrelated conference. Thanks to Andrew Steer and Mark Guiducci for comments.
[1] Strep throat would not be eradicated by a vaccine; I merely hope it is greatly reduced. Strep throat can be caused by strep C and G bacteria too, though that is currently less common. Plus, even if strep A were the only culprit, no strep A vaccine will be perfect.
[2] The new crop of funders of strep A vaccine development over the last five years includes the Australian and New Zealand governments, CARB-X, the Leducq Foundation, and the Wellcome Trust. This year, the Novo Nordisk Foundation’s Initiative for Vaccines and Immunity (NIVI) is getting started, partially focused on strep A. Sadly even at that new level, strep A is disastrously neglected, receiving roughly $2 of R&D funding per Year of Life Lost due to infections, compared to roughly $200 for cancer (and I think there should be more funding for cancer R&D!).
[3] I told a friend last week I was writing this post on strep A, hoping someone might make a vaccine. "Please!! I got strep throat 7 times in a row so had to get my tonsils out, and my ex once had to go to hospital because they thought he had invasive disease."
[4] For further reading, in particular related to public health, the Strep A Vaccine Global Consortium (SAVAC) has compiled a useful list of documents.
[5] Alternative titles I considered were “No more broken hearts” and “Clearing my throat”.